Research Focus
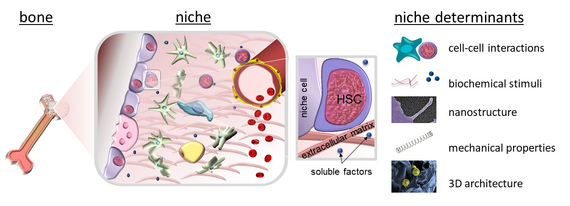
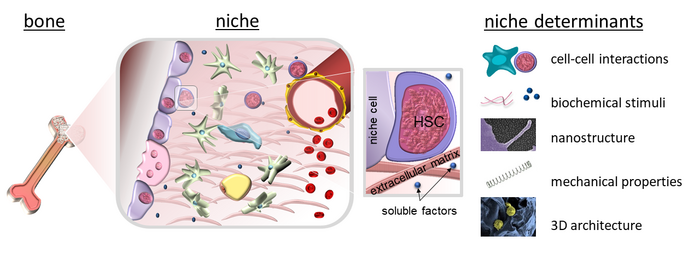
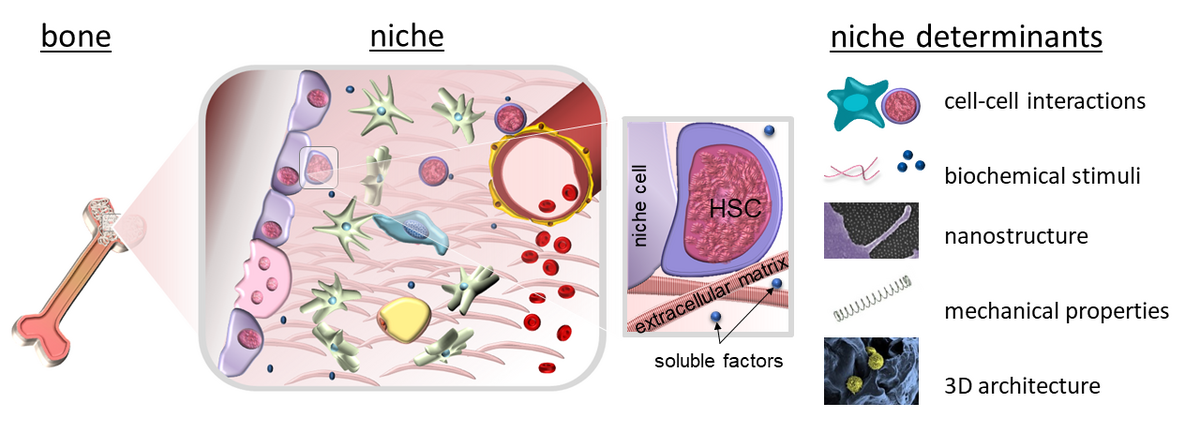
Hematopoietic stem cells (HSCs) give rise to all types of blood cells. Up to now, they cannot be expanded in vitro without loosing their stem cell character. In the body they are maintained in specialized microenvironments, so-called stem cell niches. The aim of our group is to develop and test biomaterials, which mimic the HSC niche in regard to its specific ligands, micro- and nanostructure, dimensionality as well as mechanical properties. We study the interaction of HSCs with these biomimetic materials in order to understand how the physical and mechanical properties of the environment influence HSCs. The final goal of these studies is to create an artificial stem cell niche for the in vitro proliferation of HSCs.
Projects
Blood and bone – conjoined twins in health and disease: bone marrow analogs for hematological and musculoskeletal diseases (bloodANDbone)
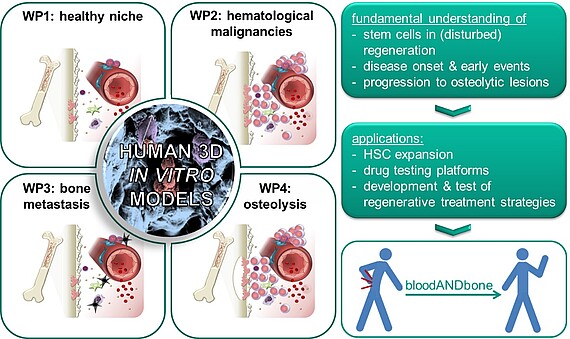

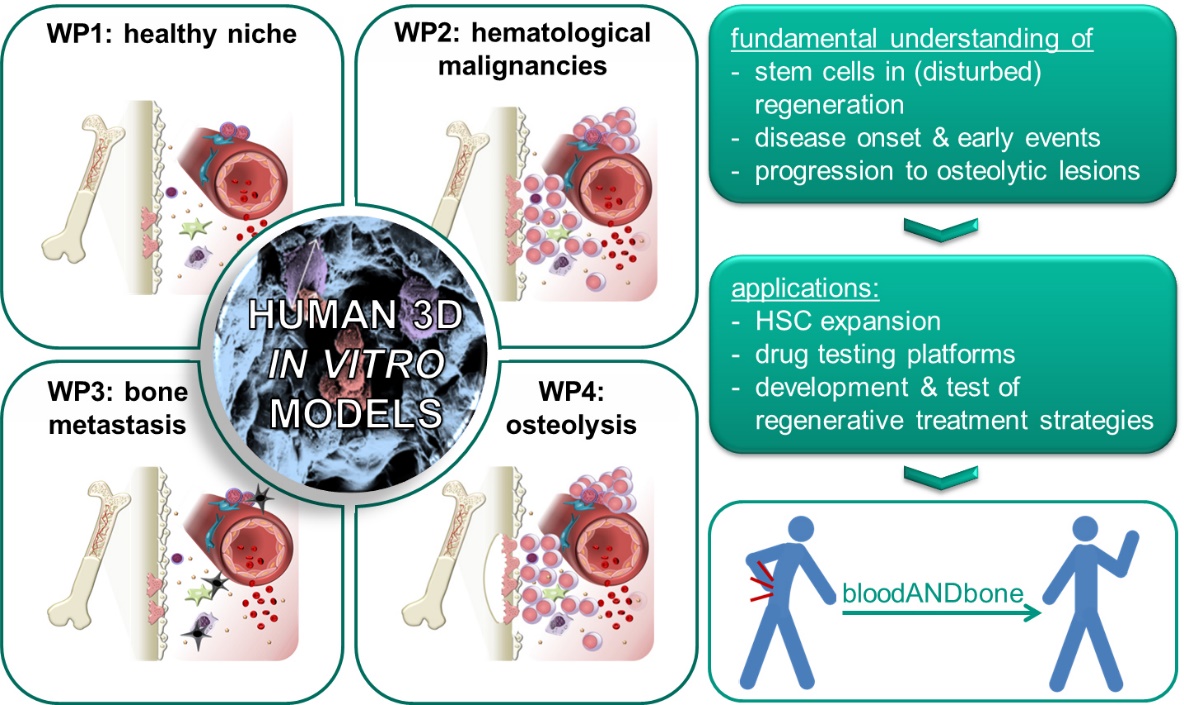
Blood and bone are closely intertwined. Their intrinsic regenerative capacities are disturbed in many hematological and musculoskeletal diseases. Re-establishing the regenerative potential is the key to cure these diseases by regenerative medicine. Multipotent stem cells of both tissues – hematopoietic stem cells (HSCs) for blood and mesenchymal stem/stromal (MSCs) for bone – are the basis for their regenerative capacity. While it is well established that HSCs are influenced by the bone marrow in their natural environment including MSCs and their progeny, surprisingly little attention has been paid to the reciprocal relationship. The hypothesis of the ERC Starting Grant project bloodANDbone is that only when taking both tissues and their mutual crosstalk into account, we will be able to understand how the regenerative potential of blood and bone is impaired in disease and how it can be re-established with novel treatment strategies. For this purpose we need to understand the early events of disease onset and progression. Due to the limitations of such studies in human beings and animals, we will develop human in vitro models of healthy bone marrow, which can be induced to develop hematological and musculoskeletal diseases with high incidence, namely leukemia, multiple myeloma and bone metastasis. Previously my team and I developed a simplified bone marrow analog that bases on macroporous, cell-laden biomaterials with tunable physical, biochemical and biological properties. This versatility will enable us to create biomimetic human in vitro models of the human bone marrow in health and disease, which will allow us to investigate how the regenerative balance of bone marrow is maintained in health and disturbed in the different kinds of diseases – a prerequisite to develop novel regenerative treatments – and to establish in vitro test systems for screening of novel drugs or treatments.
A 3D-printable biomimetic bone regeneration material (PRIOBONE)
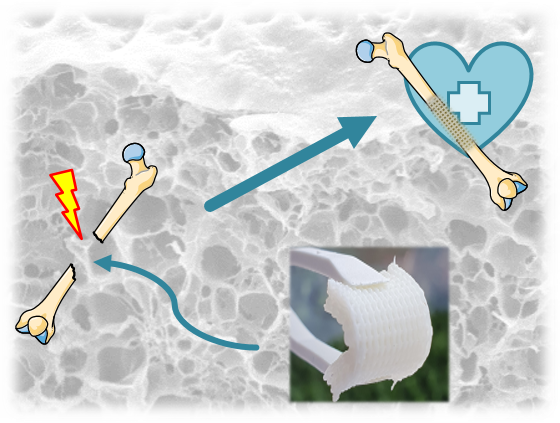
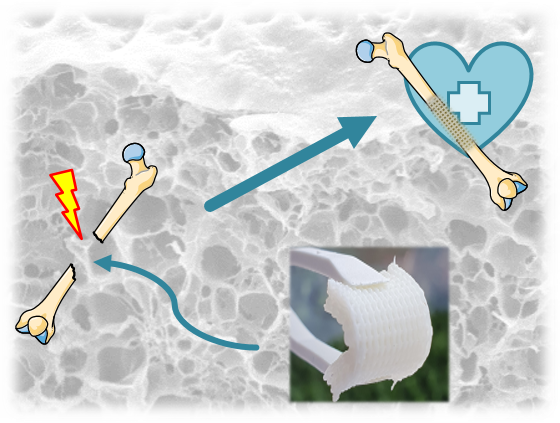
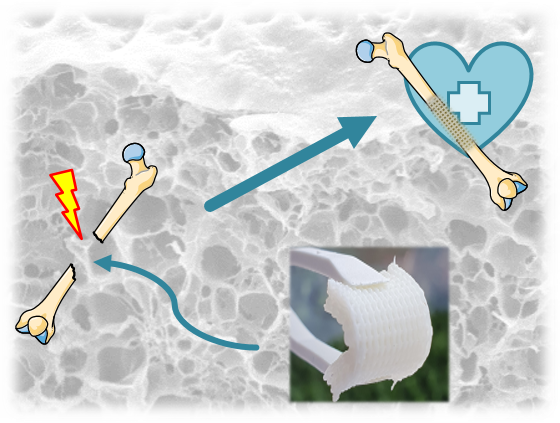
Bone defects of critical size do not heal spontaneously during the patient's lifetime and represent a significant individual, societal and economic burden. Current treatment options are hampered by associated complications, poor functional or aesthetic outcomes, limited availability of tissue for bone grafting and high financial costs. Worldwide, more than 4 million operations per year require bone grafts or replacement materials. Consequently, there is a significant clinical and economic need for novel treatments for bone defects of critical size. In the PRIOBONE project, funded by the European Research Council under a Proof of Concept Grant, we are validating a newly developed, bone-mimetic, 3D printable material for bone regeneration applications. Our PRIOBONE material can chemically and structurally mimic bone, is excellently cell-compatible, allows the differentiation of bone cells, has adjustable mechanical properties and can be 3D-printed into any desired shape. This enables us to manufacture implants that are optimized for the clinical and individual needs of patients. This includes, for example, the ability to print the material in foldable and unfoldable 3D designs that allow minimally invasive insertion of the material into defect sites where it can expand again. The use of established components and a materials-only approach allows for a faster path to clinical application and regulatory approval compared to approaches involving biologics such as cells or previously unknown components. Following successful validation of the material, we expect that PRIOBONE can provide a cost-efficient, individualizable alternative to current treatments with the potential to significantly lower the economic, individual and social burden of critical-size bone defects.
Matrix Evolution - Hierarchically structured, bio-inspired matrices
Biomaterials play a key role in regenerative medicine applications. The biophysical and biochemical properties of materials create specific cellular environments that significantly influence cell behavior and function. A wide range of natural and synthetic materials are currently being used or researched for use in regenerative medicine (tissue engineering) and implant research. Although these biomaterials already meet important requirements such as biocompatibility, degradability or mechanical stability, they are ultimately often highly simplified models of the extracellular matrix (ECM). The hierarchically structured, highly compartmentalized and dynamic complexity of the real ECM is far from being achieved by using unstructured biomaterials. The in-vitro models made from currently available biomaterials thus remain highly simplified and cannot reproduce the full biological function of tissue. There is therefore an urgent need for biomaterials that exhibit hierarchical structuring and complexity like the natural matrix and enable the construction of defined architectures from the nano to the 3D macro level. The natural ECM is not only highly structured, but also highly dynamic and is remodeled and adapted in physiological processes. Therefore, the Matrix Evolution project also aims to emulate this dynamic aspect by introducing bioresponsive and temporally switchable elements into the biomaterials. New, bio-inspired matrix molecules and materials can lead to an evolution of the matrix in tissue modeling, with significant benefits for all areas of regenerative medicine and clinical research.
Within the framework of this collaborative research project, the Cell Biology Department's "MatrixNiche" sub-project will mimic the ECM of bone marrow and the structure of the surrounding bone by combining different matrix molecules in a hierarchically organized, biomimetic biomaterial produced by 3D printing, electrospinning and the cross-linking of polymers to form hydrogels. This customized matrix will allow the combined influence of different parameters - such as nanostructure, macrostructure, stiffness and porosity - on bone marrow stem cells to be studied using high-resolution light and atomic force microscopy, single cell force spectroscopy and modern biochemical, cell and molecular biological methods. The hierarchically structured matrix is further used as a scaffold structure for a biomimetic in vitro model of the bone marrow stem cell niche composed of multiple cell types, which allows the analysis of cellular interactions depending on matrix properties in the niche.
Completed Projects
BioNanoHSC
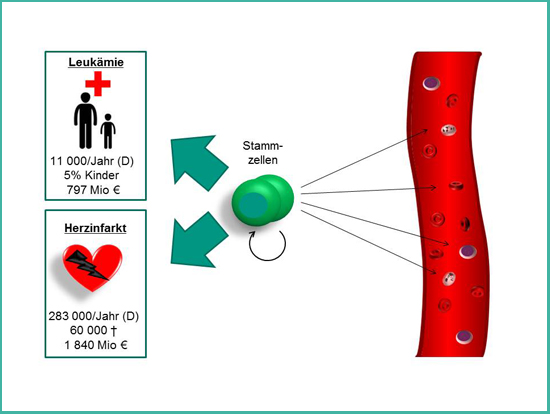
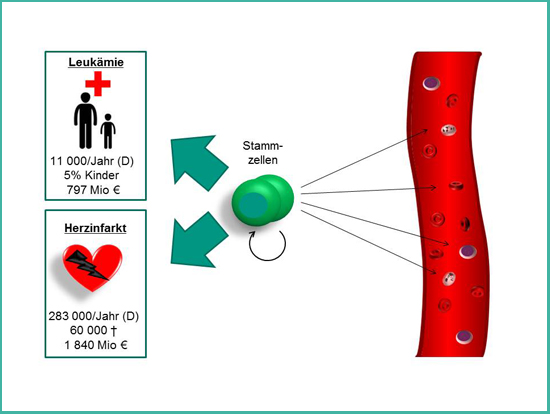
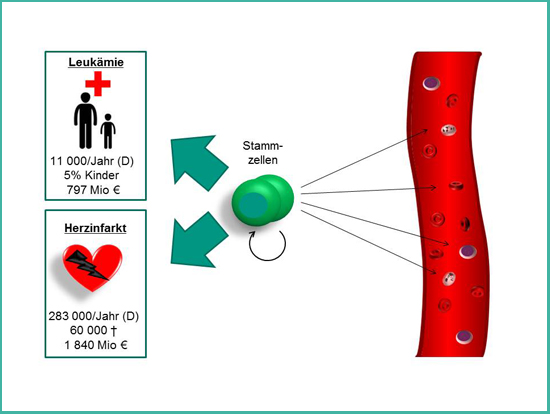
Figure 1: The stem cells of the blood. Hematopoietic stem cells are the life-long source of fresh blood cells. Since decades they are used to treat patients with hematological disorders such as leukemia. Additionally, they are promising for the treatment of patients after myocardial infarction.
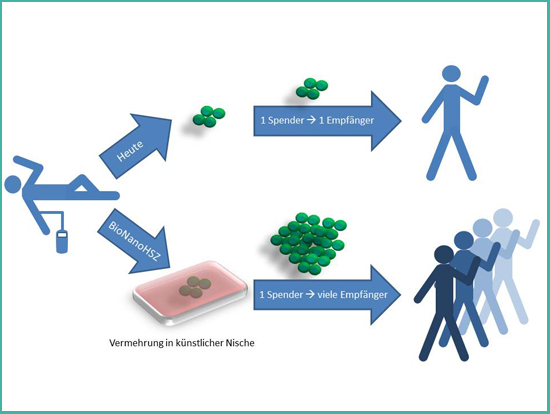
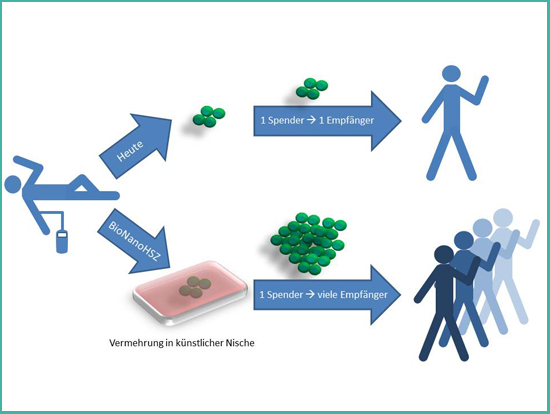
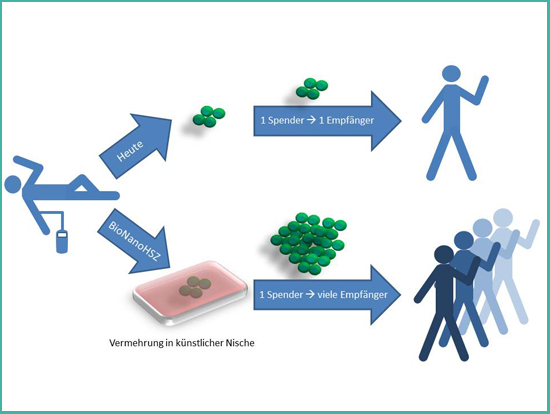
Figure 2: Principle of the project BioNanoHSC.
The project BioNanoHSC is funded by the NanoMatFutur program of the German Federal Ministery of Education and Research (BMBF) (http://www.bmbf.de/foerderungen/16771.php) with 3.18 million € (FKZ: 13N12968). The acronym BioNanoHSC stands for “Biomimetic Nanostructured Materials for Culturing Hematopoietic Stem Cells - BioNanoHSC”.
Hematopoietic stem cells are the stem cells of the blood system and supply us during our entire life with fresh blood cells. Diseases of the blood system such as leukemia can be treated since the 1960s by replacing the diseased blood system with a healthy one by transplantation of stem cells or bone marrow from a healthy donor. Furthermore, hematopoietic stem cells hold great promise for the future treatment of patients after myocardial infarction – one of the leading causes of death in Germany.
An elegant approach to cover the demand of all patients who would profit from a treatment with hematopoietic stem cells is the multiplication of these stem cells in the laboratory. Today, this is not possible with a satisfying outcome. The only place where hematopoietic stem cells can multiply without restriction is their natural environment – their niche in the bone marrow. This niche is uniquely specialized for maintaining stem cells. New scientific discoveries show that material properties of the environment have a profound impact on cells. Therefore, the aim of the project BioNanoHSC is to study how stem cells interact with innovative nanostructured materials. The gained knowledge will be used to develop new materials with tunable material properties that mimic the natural environment of stem cells. These artificial niches will help to achieve the goal of a targeted multiplication of hematopoietic stem cells, which would be a great benefit for tens of thousands of patients.
Biomaterials for the culture and targeted differentiation of iPS cells
The induced pluripotent stem cell (iPSC) technology was discovered by Shinya Yamanaka’s lab in Kyoto, Japan. They inserted four specific genes encoding transcription factors which could convert adult cells into pluripotent stem cells. iPSCs are capable to differentiate into all adult cell types and self-renewal indefinitely. Therefore they are a potential cell source for regenerative medicine applications and in-vitro disease models. To date, culture substrates coated with Matrigel™ have been presenting promising results for iPSC culture and differentiation. Although Matrigel™ provides an improvement over standard monolayer cultures, it provides some drawbacks such as batch to batch variation and decrease of cytochromic activity of the cells. As an alternative, engineered biomaterials can be fabricated by adjusting biological and biophysical characteristics to maintain iPSCs culture.
The aim of this BMWi funded project (ZF4397302CS7) is to develop biomaterials that help to fabricate a) a fully synthetic Matrigel™-mimetic hydrogel for “feeder-cell free culture” that allows investigating the influence of biophysical and biochemical parameters on iPSCs and b) biomimetic gels that mimic on the one hand the hepatic progenitor cell (HPC) niche or the hepatocyte environment for the targeted differentiation an culture of hepatic cells.
Contact


30419 Hannover

